By Leire Sofia Rossi-Crespo
“Just use your brain”, I tell myself, “it’s that simple”. Sometimes it can be, but other times, elbows deep into a chemistry problem set, it can seem that using your brain is nearly impossible. But what does it mean to “use” your brain? Do we have absolute control over our brain’s, and by consequence our, ability via conscious commands?
As humans grow and develop, their brains do too. Through exposure and guided repetition, we can learn to speak new languages, read and write, play an instrument, master a skill, grasp theories and arguments, and do things more efficiently. To assimilate new and difficult input, the human brain must be plastic: able to readily adjust and adapt in response to information. Neural activity and plasticity are found on the communication between neuronal networks and across cerebral regions through signal cascades. The initiation of these cascades can be triggered by a “conscious command” (e.g., instructing your forearm to move in a rotary motion to stir) or a “physiological command” (e.g., release of ADH when dehydrated). For either signal, there must be messengers to transfer it.
Neurons are the brain’s principal messengers. Neuronal efficiency is directly related to the frequency of information reinforcement and the amount of information we acquire. The brain’s plasticity is determined by neuronal efficiency; a brain that is able to process information with greater efficiency, speed, and acuity is a brain that is more plastic. This processing occurs in specific regions of the brain, which control specific functions. There are six main regions of the human brain: the frontal, parietal, occipital and temporal lobes, the cerebellum and the brain stem.
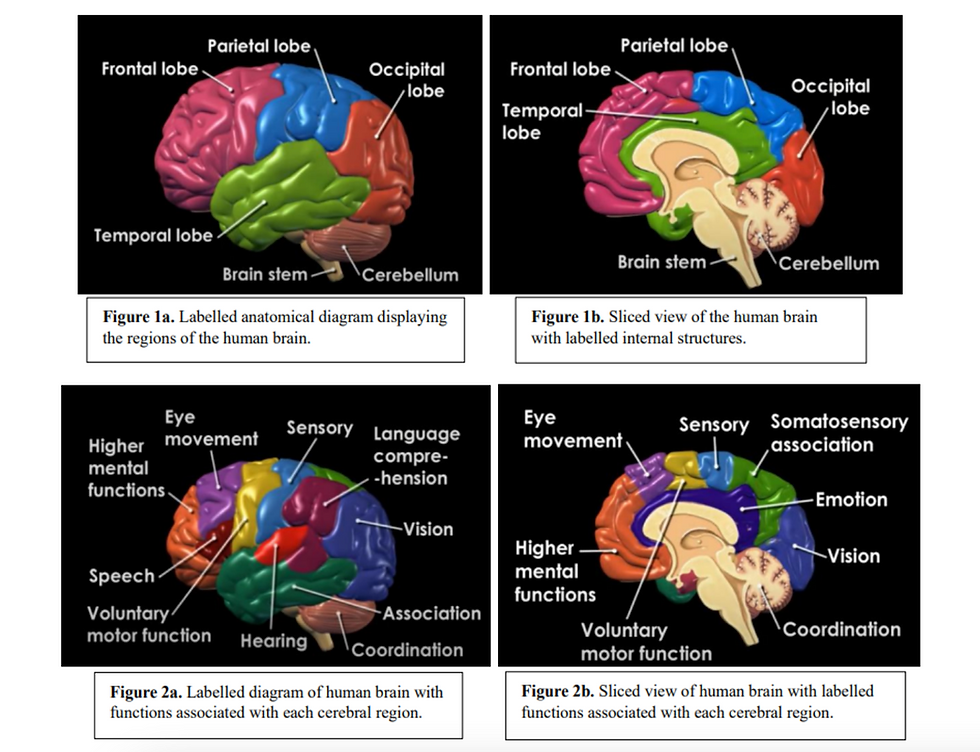
Let’s focus on the cerebellum: the center for coordination, balance and general locomotion. This region regulates the muscle control necessary to walk. Even though walking, a learned skill, is now automatic to most of us, having done it repeatedly over many years, the cerebellum still “fires up” any time we walk since the activation of its neuronal networks is required to execute the movement. The basic mechanism of neuronal communication to execute a task is described below.

What happens if there is a malfunction in this mechanism and we cannot “use” this network? Then, no signal is propagated—like a package being shipped but never reaching the mailbox. This is the case for the brains of people who have experienced stroke, brain and/or spinal cord injury, paralysis or amputation, though these each manipulate the mechanism differently.
Synaptic plasticity—the ability to use the messaging centers of the brain—is influenced by repetition and *potentiation. Long-term potentiation (LTP) describes the strengthening of synapses by lowering the stimulation threshold, thereby making communication faster and more efficient. This is the principle at the basis of learning a new skill; it may initially be challenging but it becomes easier after continuous repetition.
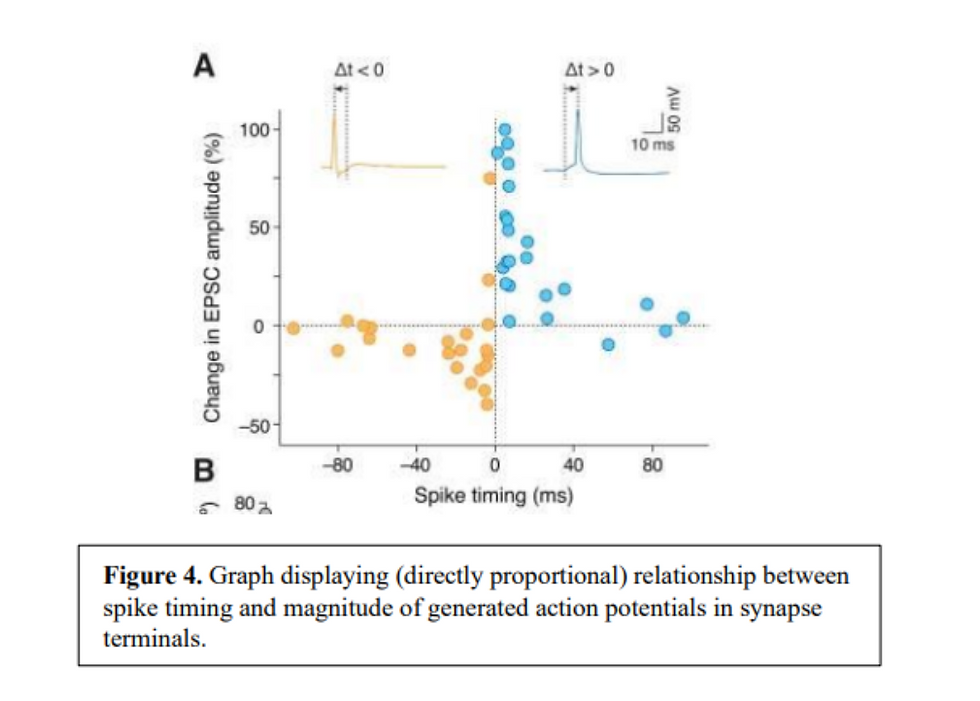
Synaptic “fitness” is dependent, among other factors within the synaptic network environment, on the timing of action potentials. Timing dependence can be exploited to regulate synaptic communication. Indeed, appropriately timed neuromuscular stimulation has been used to induce neural plasticity and achieve functional recovery from motor disorders. Many neuroprosthetic devices and machine learning interfaces, which primarily use *electrodes to discern neural signals, have made use of this relationship between synaptic stimulation and neurorehabilitation to help patients regain function following injury (Note: There are several types of neuroprostheses depending on the specific area of the nervous system that is being stimulated and the function it aims to restore, see Figure 6). Numerous studies involving single-neuron trigger sources have demonstrated the benefit of timing pre- and post-synaptic activity in the generation of plastic changes.
The simultaneous “willed” (i.e., consciously commanded) movement by the subject and electrical simulation has been proposed as a promising avenue for promoting recovery after neurological injury. Various devices that use the principles of neural interface technology have been developed over the past few years and research is still underway. The consideration of different electrodes has also been addressed to customize accordingly for certain neurological disorders or neuron-target types.

The Brain-Machine Interface (BMIs) device is an example of a neuroprosthesis that relies on electrodes to decode the subject’s neural activity. The basic mechanism by which this device works is the following: the neural signals from the subject’s brain are inputted into the interface machine, then translated into a series of locomotive movements, and finally executed by the robotic prosthetic device. Motor-based functional electrical stimulation (FES) is another technique that uses electrodes to stimulate nerves and generate muscle contraction, thereby restoring motor function.
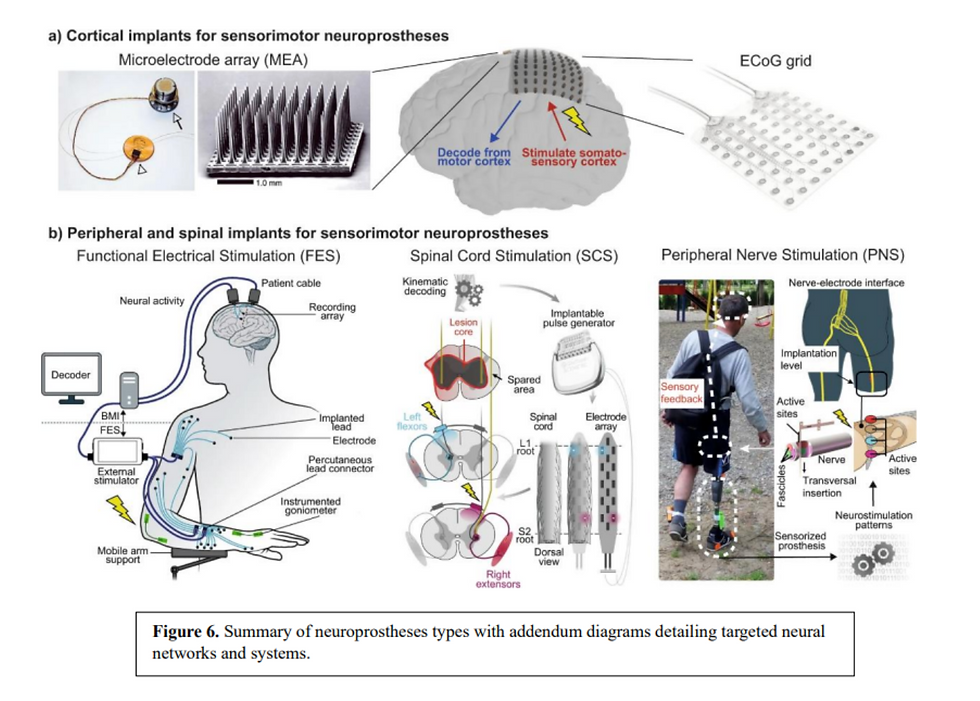
The human brain is incredibly complex and continuously generating new neurons and new neural networks. This plasticity, which we can harness and foster to develop new skills and master those to which we have already been introduced, is at the core of the brain’s ability and power. Understanding the mechanisms underlying neuroplasticity with specific regard to optimizing brain recovery and function can direct scientists to apply them for medical rehabilitation. One such case is the development of neuromuscular prosthetics that can aid patients with previous brain injury, amputation or loss of proper locomotion in regaining compromised neural ability.
*potentiation (in simple terms): stimulation of synapses.
*electrodes conduct electricity.
References:
1. Ethier, C., Gallego, J. A., & Miller, L. E. (2015). Brain-controlled neuromuscular stimulation to drive neural plasticity and functional recovery. Current opinion in neurobiology, 33, 95–102. https://doi.org/10.1016/j.conb.2015.03.007
2. Puderbaugh, M. & Emmady, P. D. (2023, May 1). Neuroplasticity. In StatPearls. StatPearls Publishing, Retrieved March 10, 2024 from https://www.ncbi.nlm.nih.gov/books/NBK557811/
3. Collinger, J. L., Foldes, S., Bruns, T. M., Wodlinger, B., Gaunt, R., & Weber, D. J. (2013). Neuroprosthetic technology for individuals with spinal cord injury. The journal of spinal cord medicine, 36(4), 258–272. https://doi.org/10.1179/2045772313Y.0000000128
4. Gupta, A., Vardalakis, N. & Wagner, F.B. Neuroprosthetics: from sensorimotor to cognitive disorders. Commun Biol 6, 14 (2023). https://doi.org/10.1038/s42003-022-04390-w
5. Ludwig, P. E., Reddy, V., Varacallo, M. (2023, July 24). Neuroanatomy, Neurons. In StatPearls. StatPearls Publishing, Retrieved March 10, 2024 from https://www.ncbi.nlm.nih.gov/books/NBK441977/
Comments